Researchers at the Georgia Tech Research Institute (GTRI) are using additive manufacturing techniques to create unique waveguide structures that would be difficult or impossible to make using conventional fabrication processes. The new techniques are especially useful for integrating updated components into equipment that might otherwise require significant design changes.
In high-powered millimeter wave and microwave radars and antennas, waveguides direct electromagnetic energy from one component to another inside the equipment. Until recently, the waveguides had been fabricated from extruded copper or aluminum tubing, but these traditional manufacturing techniques can’t always accommodate the complex configurations needed for optimal design with minimal energy transmission losses.
“To propagate electromagnetic waves efficiently, the waveguide must have a very precise internal geometry,” explained GTRI Senior Research Engineer Kyle Azevedo. “Yet, the waveguide must also be very smooth in terms of its internal surface finish. The cavity that transmits the energy has to be very well controlled to avoid significant losses. And the waveguides must also fit into confined spaces.”
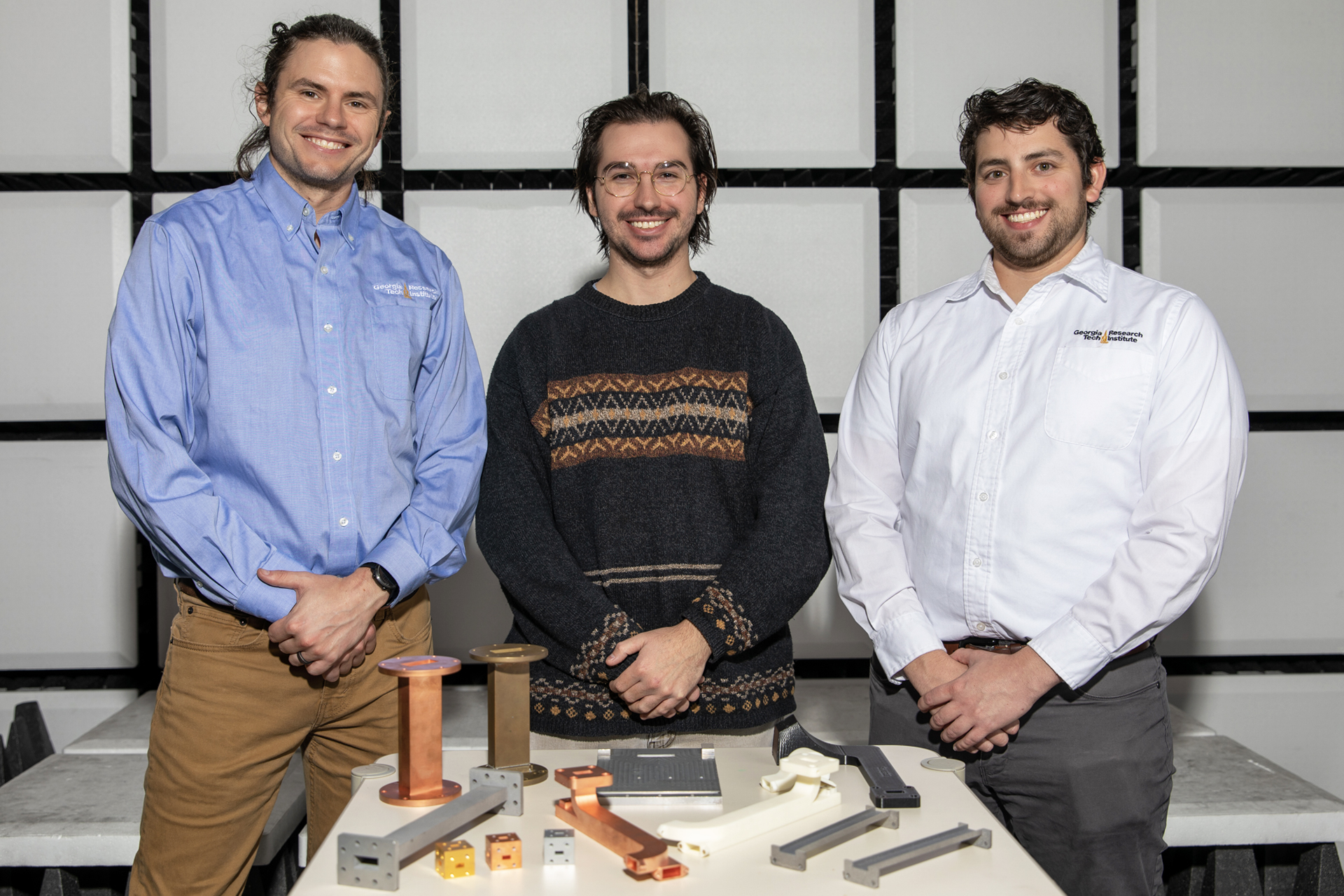
GTRI researchers Kyle Azevedo, Austin Forgey, and Max Tannenbaum are shown with examples of additively-manufactured waveguide components. Photo credit: Sean McNeil
Additive Techniques Offer Specific RF Advantages
To allow more complex designs, GTRI researchers are evaluating two alternative fabrication techniques: 3D-printed structures fabricated from metal and 3D-printed polymer components that are metal coated. Each has advantages and disadvantages and must be chosen for the specific application. The researchers are testing waveguides made using both techniques for mechanical performance in resisting fatigue damage, thermal performance in dissipating heat, electrical performance – and their RF energy loss.
Flexibility afforded by additive techniques can help designers accommodate waveguides within a crowded design that includes many other components. In one current project, the researchers found that they needed to move a radar’s feed horn several inches to accommodate other design considerations. But that complicated the waveguide design.
“That was a really big challenge, because we had to change some of the existing waveguides, and we couldn’t do that with traditional tubing pieces that were available without coming up with a whole new design,” Azevedo said. “But by using additive processes that allow more customization, we were able to make things smaller and optimize the design in a single iteration.”
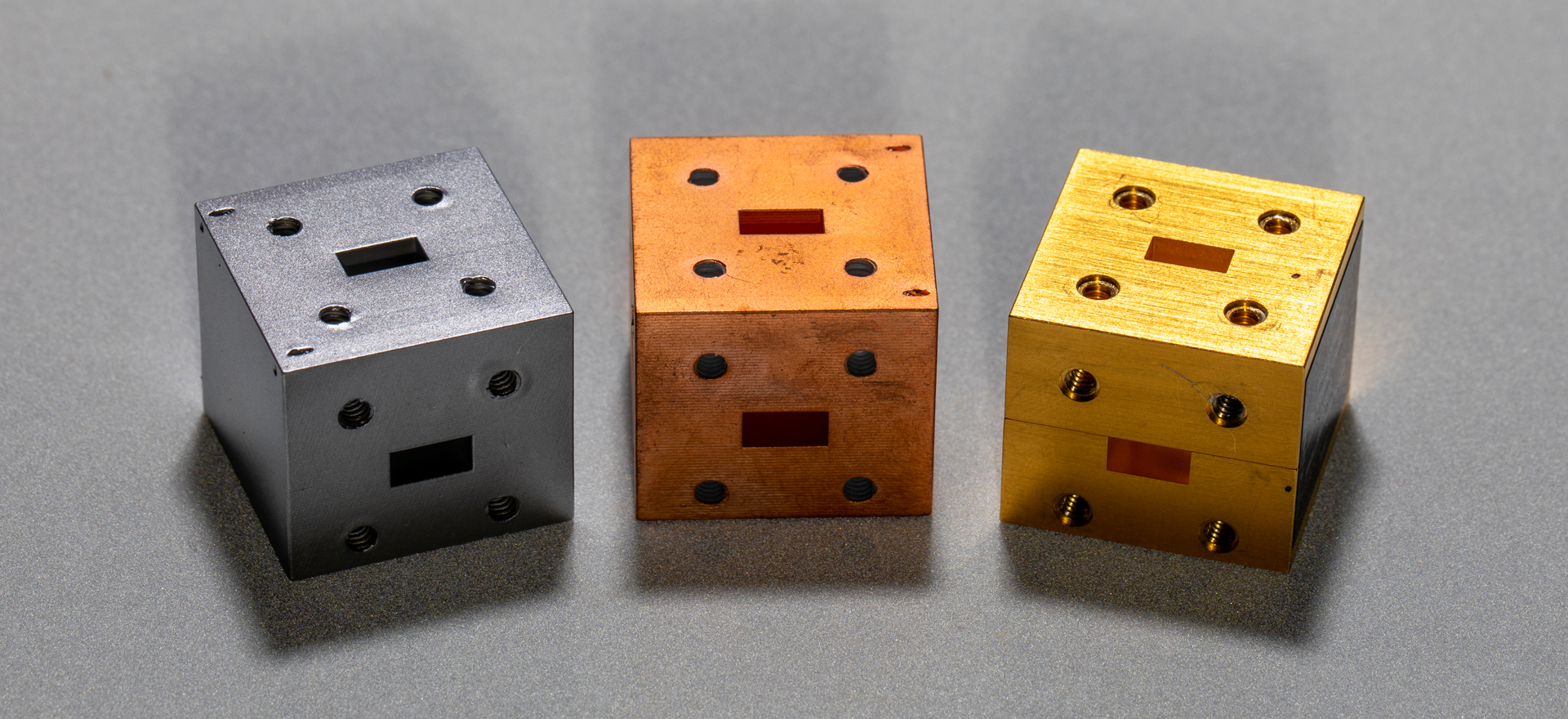
“Magic Tee” components fabricated and tested to validate the performance of plated polymer waveguides. (L-R) A copper-plated component covered with an aluminum finish, a copper-plated version without aluminum, and a brass COTS structure for comparison. Photo credit: Sean McNeil
In another project – in consideration for use on Army counter-battery radar – researchers at U.S. Army DEVCOM C5ISR Center used additive techniques to accommodate an updated component of a different size and shape than the original to improve RF performance. The researchers designed a 3D-printed waveguide that accommodated the existing connectors and integrated into its legacy systems, then worked with the Army’s Rock Island Arsenal to optimize fabrication and quality control. That new component was successfully tested at Tobyhanna Army Depot and Yuma Proving Ground, and is now being evaluated as an OEM alternative. This may allow additive manufacturing to augment the supply system.
In addition to facilitating designs that might otherwise have been more challenging, the GTRI researchers expect additive manufacturing will allow them to accelerate the iterative development of prototypes by moving some waveguide fabrication in-house.
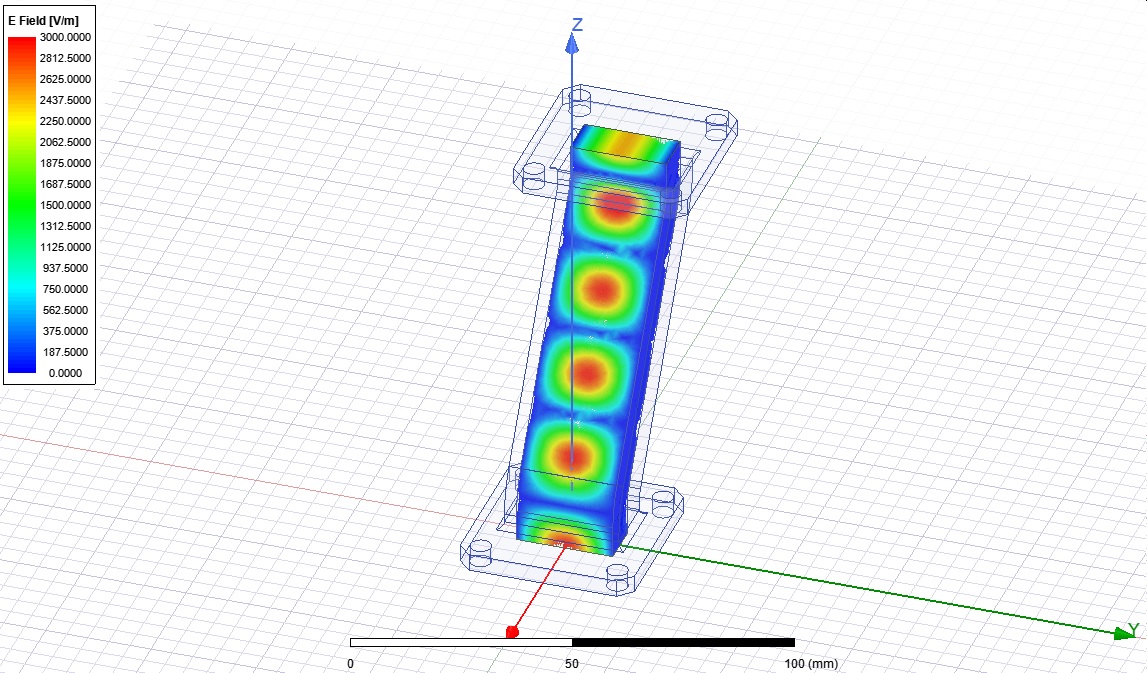
Simulation results show the efficiency of wave propagation in a proposed waveguide design. GTRI researchers use advanced modeling and simulation to investigate multiple design variants before attempting any 3D-printed prototypes, then verify the performance of their analytical models once parts are produced. Image credit: U.S. Army DEVCOM
Overcoming Challenges with New Fabrication Methods
For all-metal waveguides, additive manufacturing can have some disadvantages, but those may not be as significant as they might first appear. To limit transmission losses, inside surfaces of conventional waveguides are smooth, but because of the way metal 3D printing works, smooth internal surfaces can be difficult to fabricate. The design flexibility of additive processes can make up for that.
“In one of our designs, we found that even though we might have some limitations on the roughness of the surface finish, we could gain back the transmission losses by optimizing the waveguide shape,” Azevedo explained. “The final design would not have been possible using traditional waveguide fabrication techniques.”
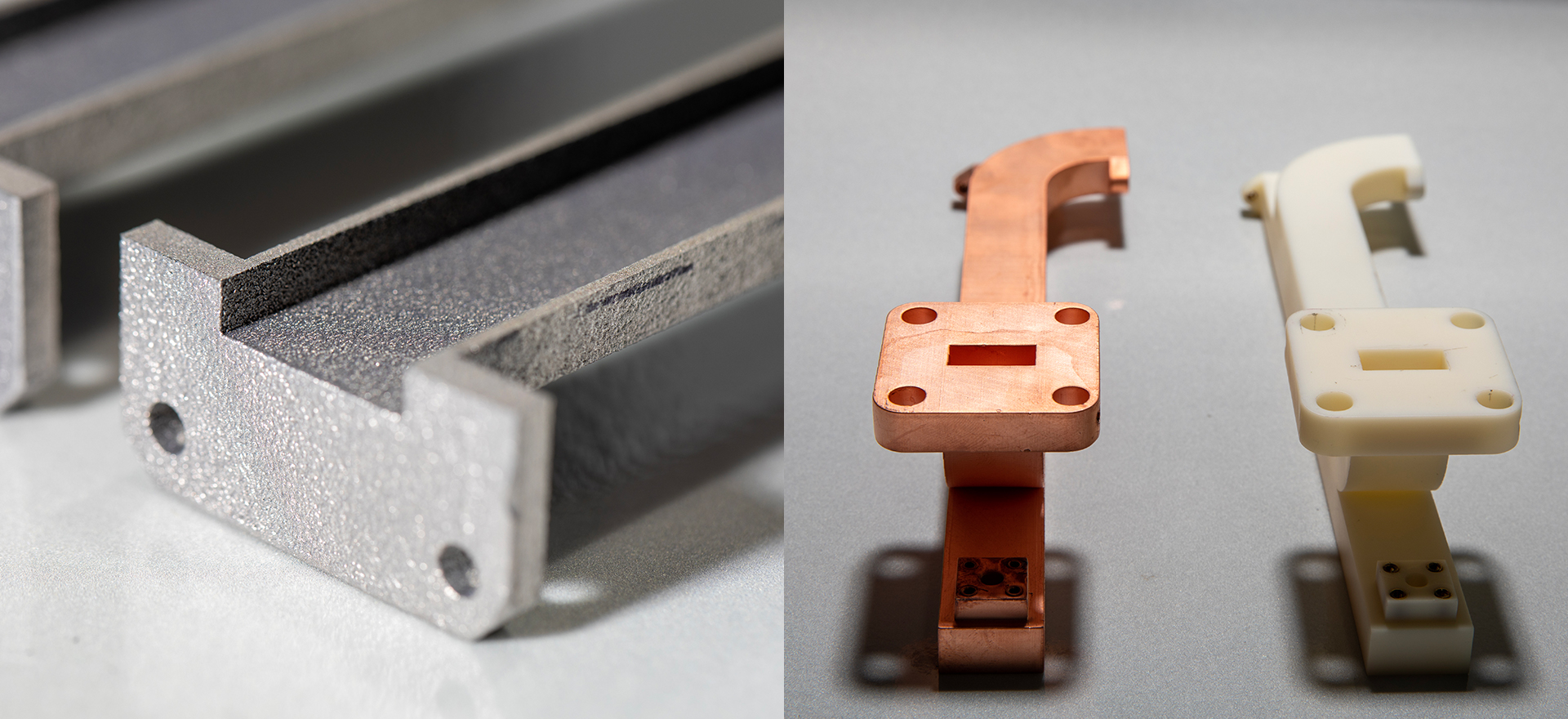
Left: A straight section of metal 3D-printed Ku-band waveguide. Metal 3D printing has advantages, but at higher frequencies, transmission loss in waveguide components is often an issue due to increased surface roughness. Right: Side-by-side view of waveguide components with and without copper plating. The polymer used for these applications is a silica-filled ceramic-like material printed using a stereolithography process. Photo credit: Sean McNeil
Though applying metal coatings to waveguides produced from polymers or resins can provide smoother surfaces, this process comes with its own set of challenges.
“One of the issues we are tackling now is that a lot of the resins and polymers that have desirable properties for plating contain silica,” said Max Tannenbaum, a GTRI research engineer. “They are ceramic-like when cured, but when you remove the resin, a lot of the conventional solvents don’t remove the silica, and you end up with a chalky powder on the surface. If you can’t remove that before you try to plate it, the plating won’t adhere.”
Building a Knowledge Base for RF Applications of Additive Processes
To support expanded applications for waveguides fabricated using additive techniques, the researchers are using both simulation and experimentation to develop comprehensive design information.
“The traditional method for making waveguides has been around since World War II, when the original systems got up and running, and those techniques have been refined over the decades since then,” said Azevedo. “As a result, there’s a wealth of detailed data on what works, including the metallurgic properties. We want to develop a comparable depth of understanding for the new additive manufacturing techniques that offer so many advantages.” By putting together what they have learned, the research team plans to share their knowledge with other RF engineers who may be interested in the additive approaches.
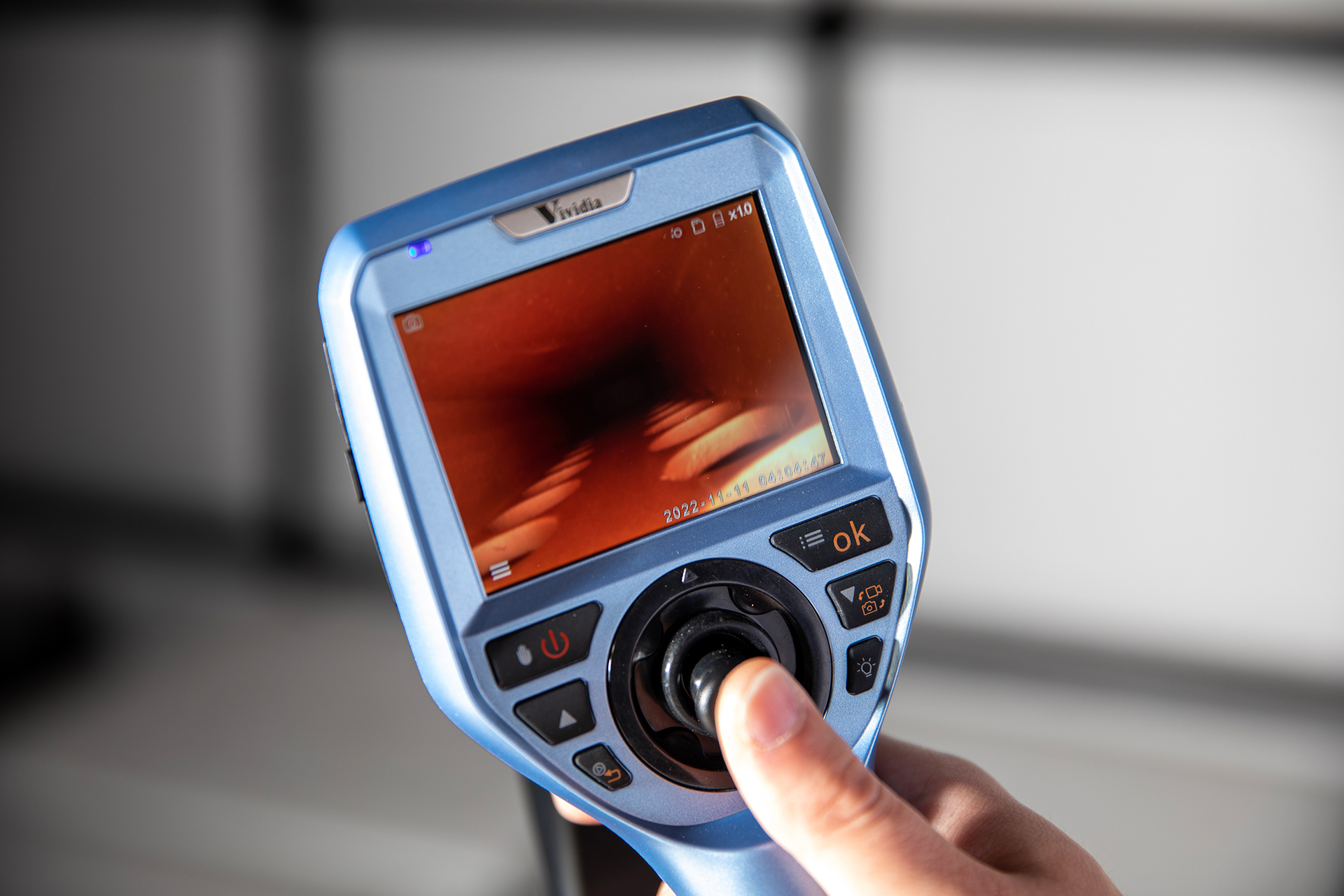
Closeup image displayed on a borescope showing some of the features that make up a broad wall coupler produced through additive manufacturing techniques. Photo credit: Sean McNeil
“Our effort is focusing on two parallel paths: looking at the mechanical constraints involved with fabrication and the RF limitations,” said GTRI Research Engineer Austin Forgey. “We are merging the new experimental data we’re getting with RF simulations, and combining that with testing mechanical properties. That will give us a full design package that can be used by the designers who need it.”
Beyond waveguides, the GTRI researchers are working on other applications of additive manufacturing to RF design. With researchers in Georgia Tech’s School of Mechanical Engineering, they are fabricating cold plates that are 3D printed from aluminum and used to cool high-powered electronics. The 3D printing allows novel fluid flow in a single part, not possible with conventional fabrication.
Similarly, they are also looking at additive techniques to make shielding needed to protect components from electromagnetic interference.
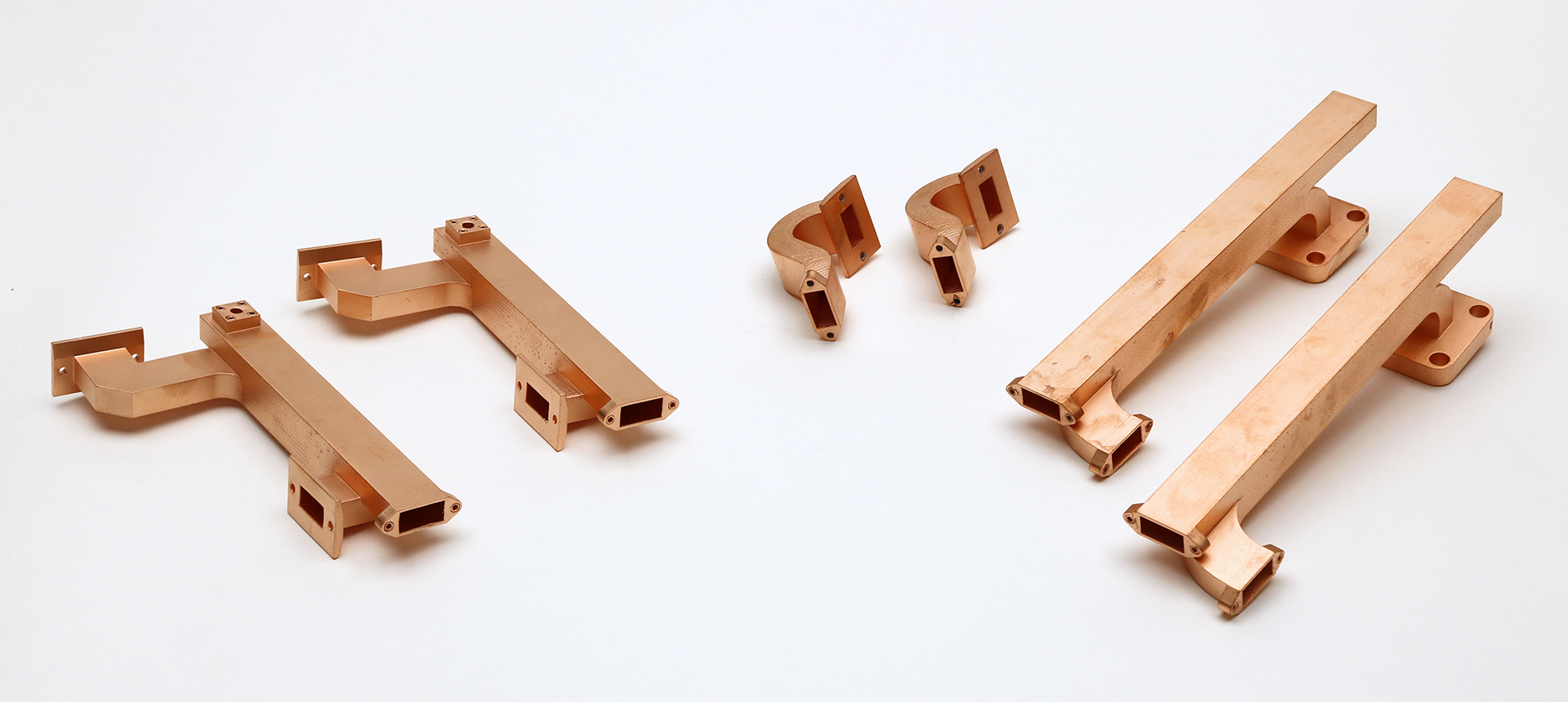
Plated polymer waveguide sets designed by GTRI for a specific application. Among the features in these parts are SMA adapters, broad wall couplers, and flanges with threaded inserts. Photo credit: Kay Lindsey
While they expect to expand their use of additive fabrication approaches to provide new design options for RF applications, the researchers don’t see additive manufacturing doing away with conventional fabrication techniques any time soon.
“My opinion is that there will certainly be a mix because additive manufacturing techniques aren’t the answer for everything,” said Tannenbaum. “But they are solutions to a lot of specific problems that we encounter, allowing us to build parts that are cheaper, lighter, and available more quickly.”
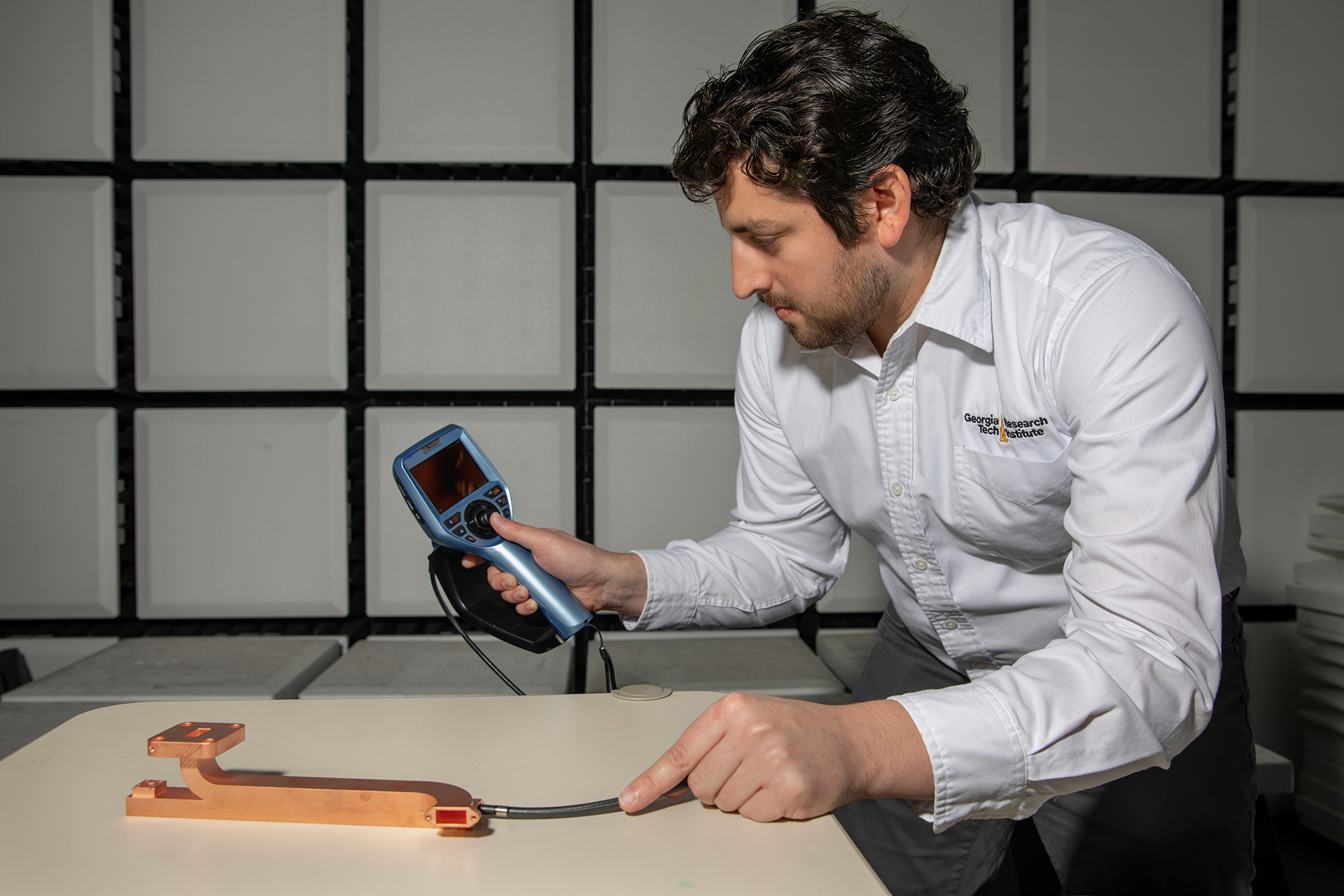
GTRI researcher Max Tannenbaum uses a borescope to inspect plating quality on the interior of a custom waveguide. Photo credit: Sean McNeil
Writer: John Toon (john.toon@gtri.gatech.edu)
GTRI Communications
Georgia Tech Research Institute
Atlanta, Georgia USA
MORE 2022 ANNUAL REPORT STORIES
MORE GTRI NEWS STORIES
The Georgia Tech Research Institute (GTRI) is the nonprofit, applied research division of the Georgia Institute of Technology (Georgia Tech). Founded in 1934 as the Engineering Experiment Station, GTRI has grown to more than 2,800 employees supporting eight laboratories in over 20 locations around the country and performing more than $700 million of problem-solving research annually for government and industry. GTRI's renowned researchers combine science, engineering, economics, policy, and technical expertise to solve complex problems for the U.S. federal government, state, and industry.